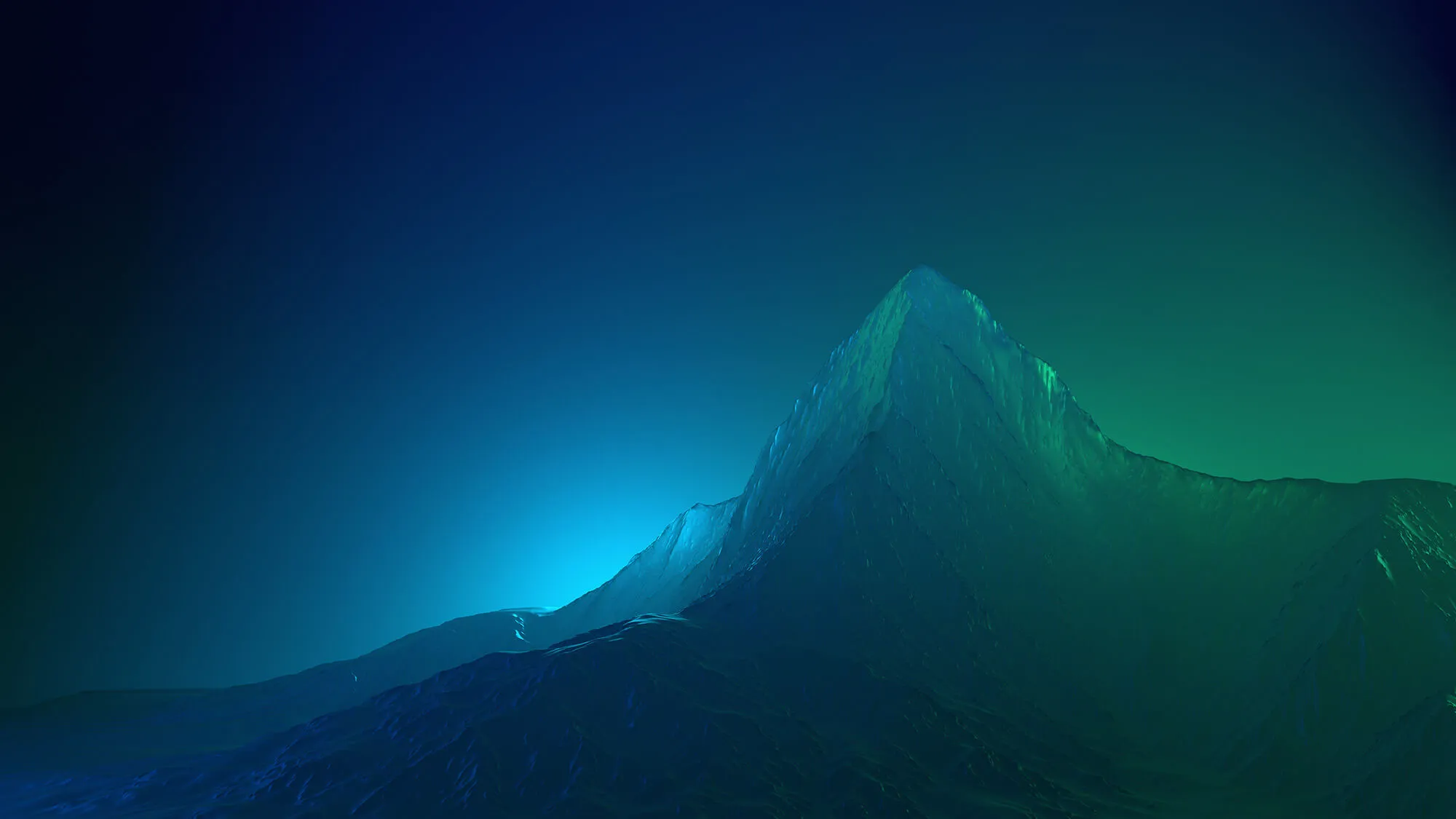
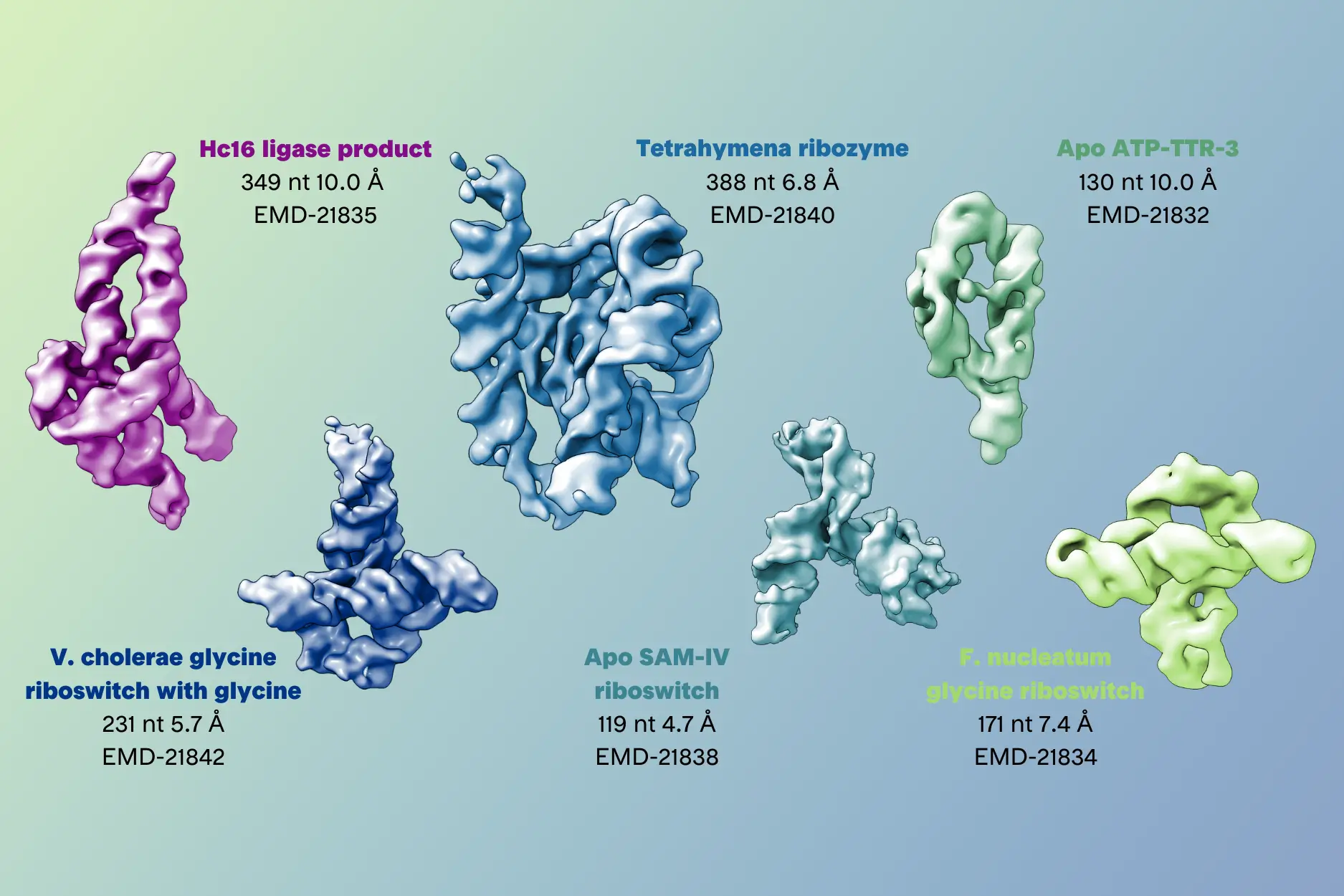
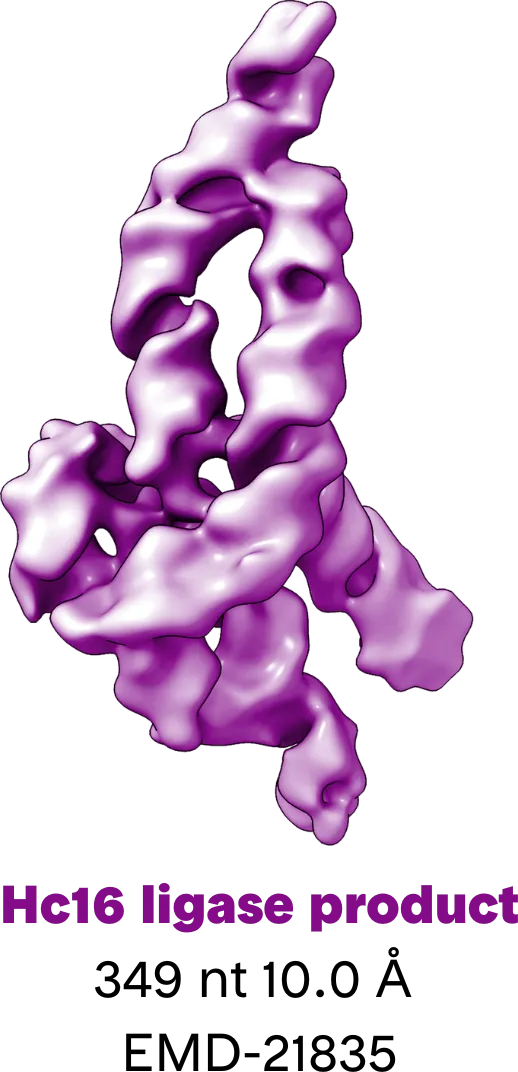
The RNA Revolution
RNAs are versatile molecules that perform a wide range of functions within the cell, including information transfer, catalysis, gene regulation, structural roles, and more, often without the need to bind to proteins. This versatility makes RNAs multifaceted players in cellular processes. Understanding their structure is key to unraveling the diverse functions. In particular, attention is focused on two key functions that RNA molecules can carry out independently: catalysis and gene regulation.
RNA as Enzymes
RNA molecules demonstrating enzymatic activity are categorized as ribozymes. Similar to proteins, ribozymes possess catalytic capabilities, challenging the conventional notion that only proteins could serve as enzymes. In 1982, Thomas Cech and Sidney Altman were awarded the Nobel Prize in Chemistry for their groundbreaking work in identifying this class of molecules. Ribozymes can be classified in several structural classes, such as self-splicing, hammerhead, hairpin, group I and group II introns, among others, each one with specific catalytic functions.
Ribozymes have garnered significant attention due to their potential therapeutic applications. Researchers have explored the utilization of synthetic ribozymes to target specific RNA sequences, including those within viral RNA or disease-associated mRNA. This innovative approach, known as ribozyme therapy, holds great promise in treating a wide spectrum of diseases. Continuous research and advancements in RNA engineering and delivery systems are expected to enhance the efficacy and versatility of ribozyme therapy in the future.
Regulatory RNA element
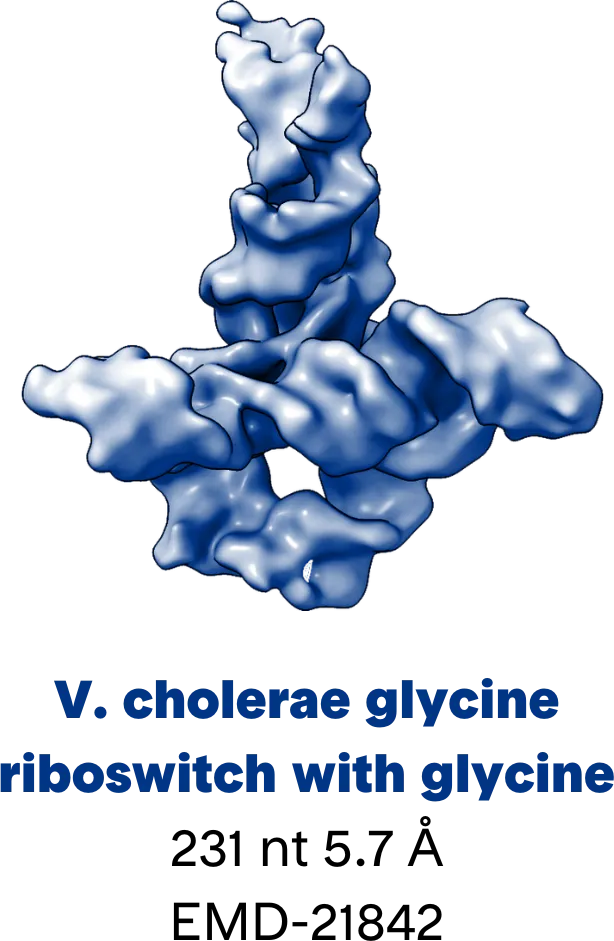
Riboswitches are regulatory RNA elements found in the 5' untranslated regions (UTRs) and non-coding regions of certain mRNA molecules. These elements play a remarkable role in gene regulation, directly controlling gene expression in response to specific molecules or environmental conditions. Typically, riboswitches consist of two primary regions: the aptamer domain, responsible for recognizing and binding to specific small molecules or ions, and the expression platform, a region capable of adopting multiple conformations depending on whether the aptamer domain is bound to its target, thereby influencing gene expression. Well-known riboswitches include thiamine pyrophosphate (TPP), S-adenosylmethionine (SAM), flavin mononucleotide (FMN), and glucosamine-6-phosphate (GlcN6P) riboswitches.
Riboswitches function as versatile and efficient mechanisms for gene regulation, allowing cells to adapt to changing environmental conditions and metabolic requirements. These elements are gaining prominence in biotechnology and synthetic biology as they can be harnessed for designing biosensors or genetic circuits.
Challenges and Solutions in RNA Structural Biology
In the past, the primary techniques employed for elucidating the structures of biomolecules were X-ray crystallography and NMR spectroscopy. However, these methods often face limitations when applied to RNA, given the inherent heterogeneity of RNA molecules. This heterogeneity arises from the flexible ribose and phosphate backbone, weak long-range tertiary interactions, the existence of alternative conformations, and the dynamic nature of RNA in multiple functional states. These challenges make it difficult to obtain high-resolution RNA crystals and to use NMR for solving the structures of RNA molecules containing more than 100 nucleotides. This is where cryo-EM steps in to provide a solution.
Cryo-electron microscopy is a structural biology technique that involves freezing samples in vitreous ice and imaging them with electrons. This method allows scientists to visualize biological molecules at near-atomic resolution, without the need for crystallization.
One of the most significant advantages of cryo-EM is its ability to study RNA in its native, unbound state, without the influence of associated proteins. Nevertheless, studying RNA structures with cryo-EM comes with its own set of challenges. As discussed above, RNA molecules are more flexible and dynamic, which can make image processing and data analysis complex. However, recent advancements in cryo-EM technology and data processing algorithms have made it possible to overcome these challenges. (see reference papers below)
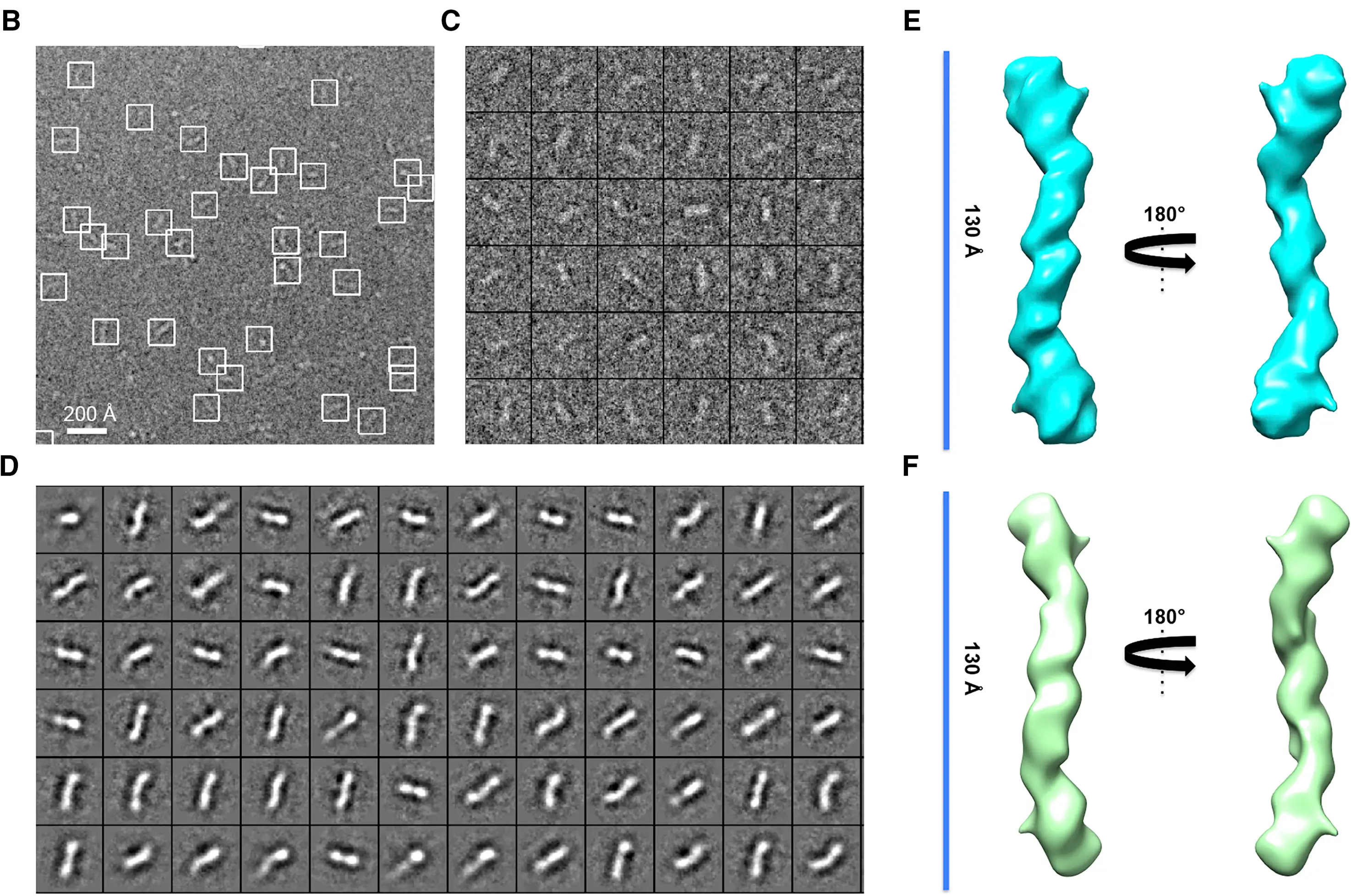
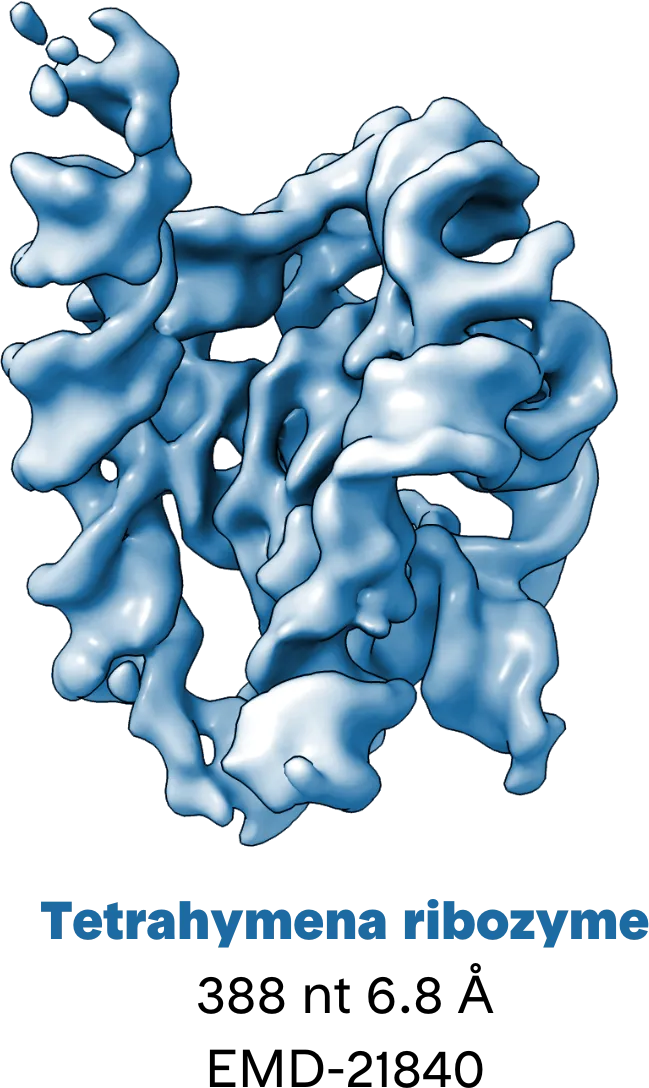
The Future of RNA Research
As cryo-EM continues to advance, we can anticipate an abundance of breakthroughs in the field of RNA research. The capacity to study RNA structures independently of proteins in their native state provides unprecedented insights into their functions and mechanisms. This not only expands our fundamental comprehension of biology but also opens doors to exciting possibilities in therapeutic interventions and drug development. The knowledge generated through cryo-EM analysis will play an important role in the development of RNA-based therapies and novel drug targets.
.webp)
Citations & References
- H. Ma, X. Jia, K. Zhang, Z. Su. Cryo-EM advances in RNA structure determination. Signal Transduct. Ther. 2022. https://doi.org/10.1038/s41392-022-00916-0
- S. L. Bonilla, J. S. Kieft. The promise of cryo-EM to explore RNA structural dynamics. J. Mol. Biol. 2022. https://doi.org/10.1016/j.jmb.2022.167802
- K. Kappel, K. Zhang, Z. Su, A. M. Watkins, W. Kladwang, S. Li, G. Pintilie, V. V. Topkar, R. Rangan, I. N. Zheludev, et al. Accelerated cryo-EM-guided determination of three-dimensional RNA-only structures. Nature Methods 2020. https://doi.org/10.1038/s41592-020-0878-9
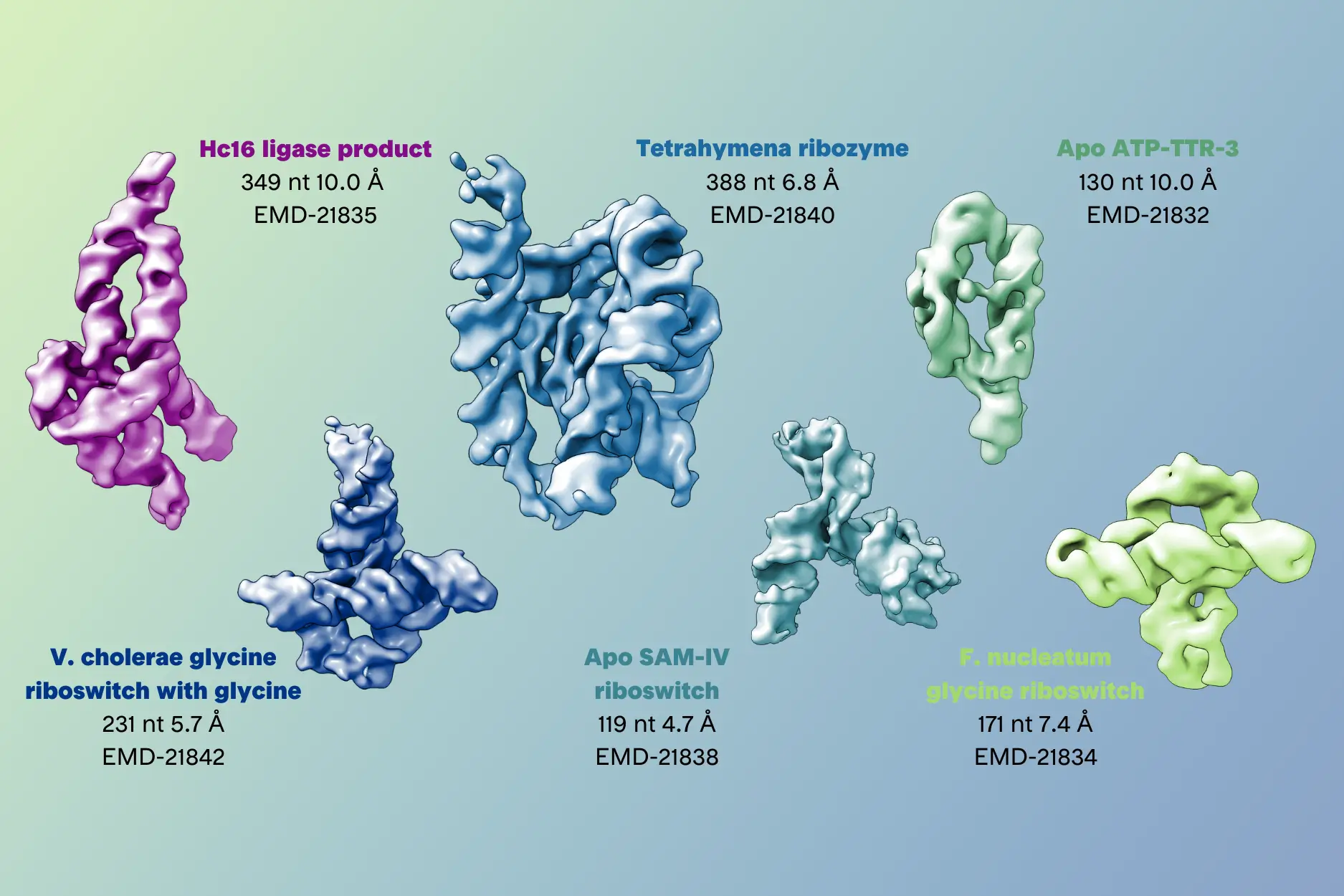
Welcome to Finsweet's accessible modal component for Webflow Libraries. This modal uses Webflow Interactions to open and close. It is accessible through custom attributes and custom JavaScript added in the embed block of the component. If you're interested in how this is built, check out the Attributes documentation page for this modal component.
Infographic Available for Download
Unlock the secrets of RNA molecules with cryo-EM. High resolution data collection & structure determination with cryo-EM for structure based drug discovery at NIS.
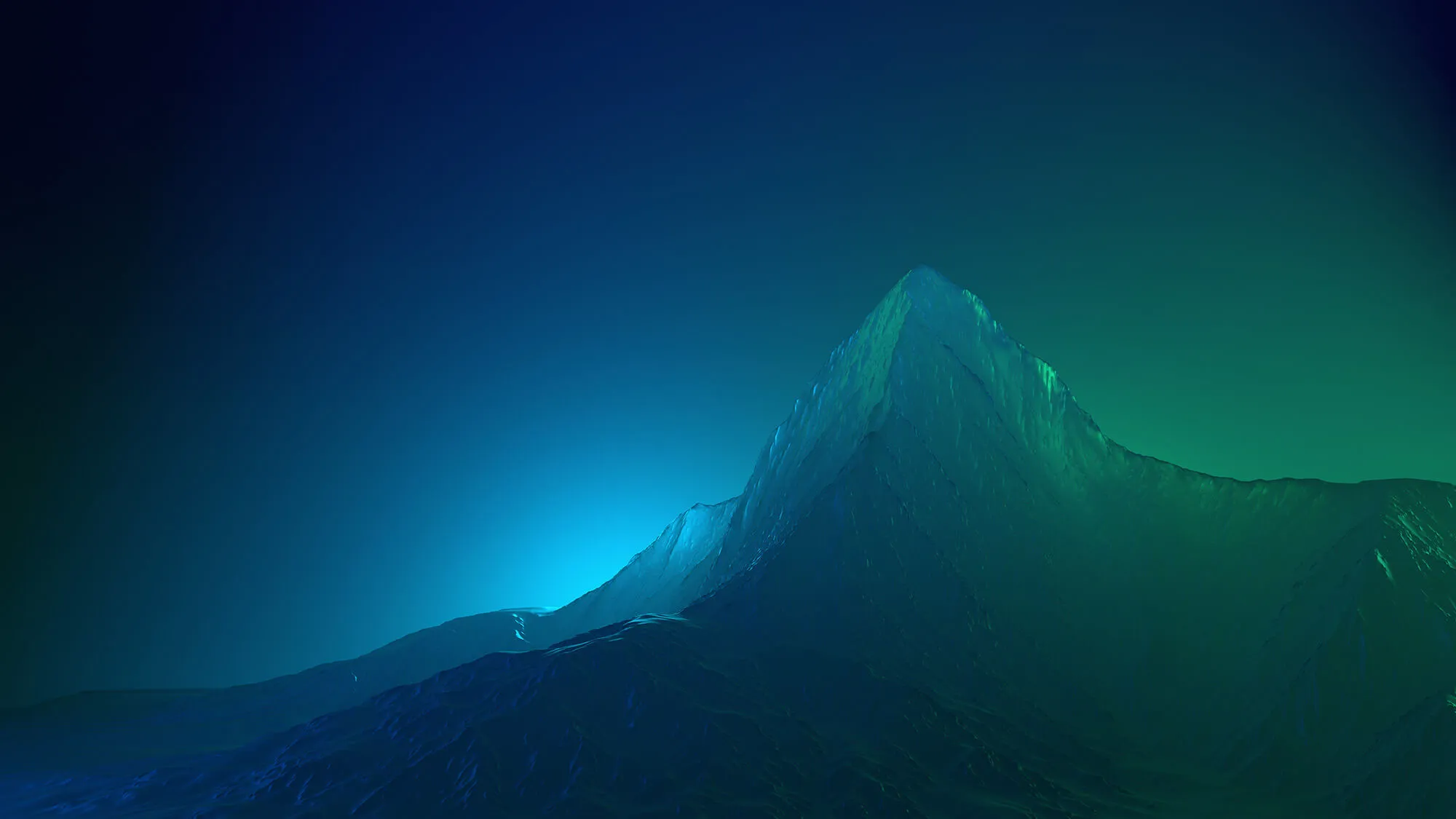