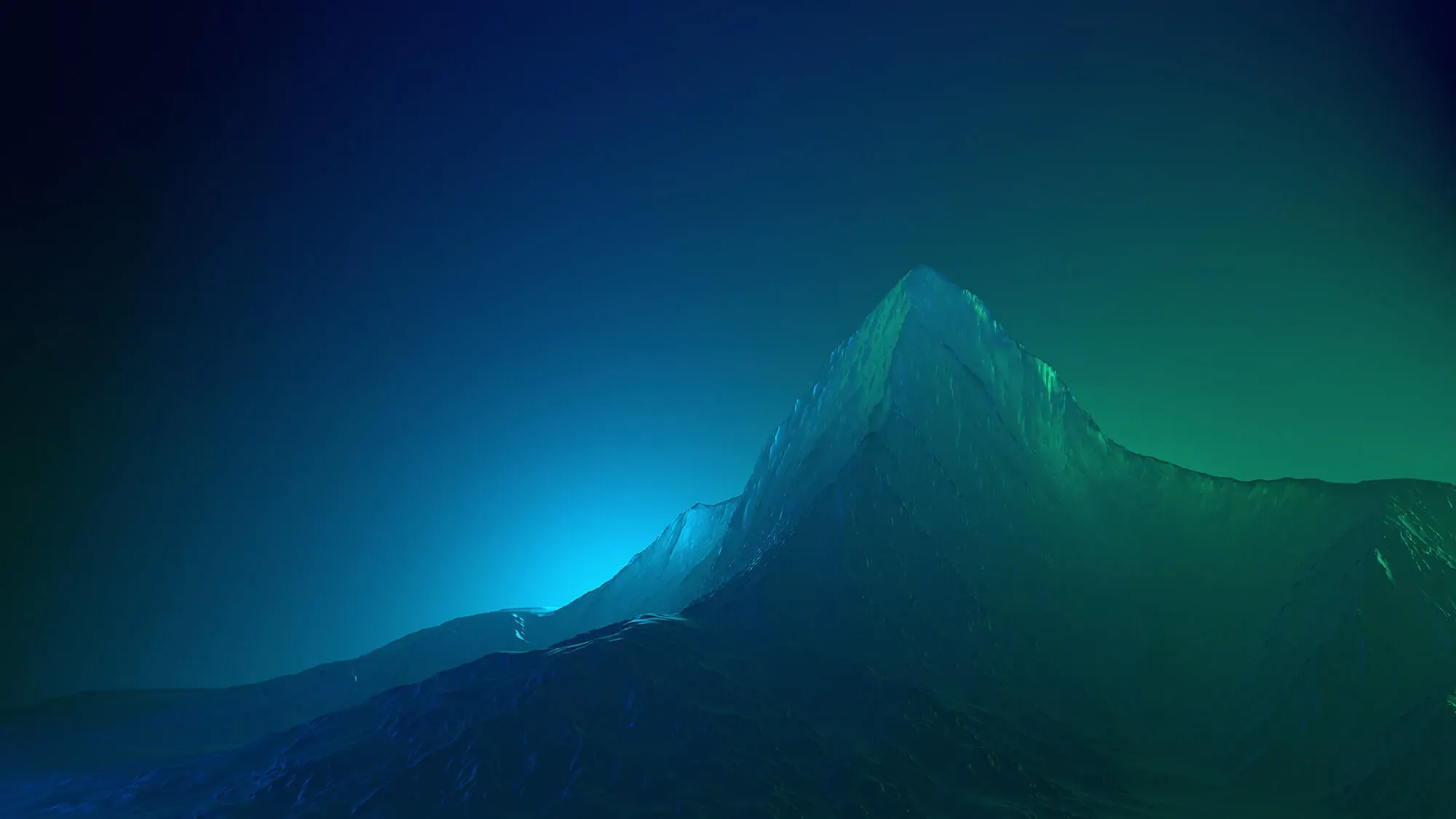
.webp)
G Protein-Coupled Receptors (GPCRs) have long been the focal point of research within the realm of molecular biology, pharmacology, and drug discovery. These versatile membrane proteins play a crucial role in transducing extracellular signals into intracellular responses, thereby regulating a wide variety of physiological processes. GPCRs are the largest family of proteins encoded in the human genome and they are also the most drugged. As of 2021, an estimated 530 drugs–representing about 30-35% of drugs available on the market—targeted GPCRs, with over 150 proteins within the GPCR family being validated as drug targets[1]. In this blog post, we will discuss the fascinating world of GPCRs from a structural biology perspective, exploring their architecture, conformational dynamics, and implications for drug development. We also provide a case study that explores how structural biology has deepened our understanding of the Glucagon-like peptide-1 receptor (GLP-1R).
Overview of GPCRs
There are six classes of GPCR, four of which are found in humans (Class A, B, C and F). Structurally, GPCRs contain a canonical fold of seven transmembrane (7TM) helices which are connected by alternating intracellular and extracellular loops. This compact structure is essential for their function of transmitting signals across membranes. Certain classes of GPCRs may have additional domains beyond this central domain, such as Class B GPCRs which often have an extracellular domain (ECD).
GPCRs are involved in the regulation of a myriad of physiological processes, ranging from sensory perception to immune response and hormonal regulation. These receptors can be activated and regulated by a wide variety of signaling molecules and drugs through a well-defined process of signal transduction that involves several key steps. In its resting state, a GPCR will bind a G𝛂 protein, which conversely interacts with a G𝜷 and G𝜸 protein as well as GDP. When a signaling molecule binds to the extracellular portion of the GPCR, a rearrangement of the 7TM bundle results in the exchange of GDP for GTP and the release of G𝛂 protein from the complex. This process initiates a signal cascade inside the cell. The release of the G𝛂 protein allows the GPCR to release the native ligand and return to a primed state where it can accept another G𝛂 protein bound to GDP (Figure 1).
.webp)
In addition to binding G-proteins, GPCRs can also interact with arrestins which are multifunctional proteins involved in desensitization, internalization, and signaling of GPCRs[2]. Therefore, arrestins are a potentially interesting therapeutic target in their own right.
Structural information and additional experimental data about specific GPCRs can be found in the GPCR Database. Recently, this database has expanded to include structure models of GPCR–G protein complexes generated using AlphaFold2, which helps predict coupling selectivity. Computational programs such as AlphaFold2 and RoseTTAfold also allow us to predict both active and inactive GPCR conformations. While these computational advancements have significantly enhanced our understanding of GPCR structures, it is important to remember that their outputs are still predictions that require validation through experimental data. Even minor discrepancies or inaccuracies in the predicted structures can have significant consequences, particularly in drug development for human use.
Structural Biology of GPCRs as a Tool for Drug Development
Structural biology techniques, such as X-ray crystallography and cryo-electron microscopy (cryo-EM), have been pivotal in capturing these dynamic changes at the atomic level. X-ray crystallography has provided high-resolution static snapshots of GPCRs in different states, while cryo-EM has enabled researchers to visualize GPCRs in complex with the trimeric G-proteins and in a variety of conformations.
Over the past few decades, a plethora of GPCR structures have been elucidated, providing a treasure trove of information for researchers. Notable examples include the beta-adrenergic receptors, rhodopsin, and the adenosine A2A receptor. These structures have unveiled the intricate details of ligand binding, receptor activation, and interactions with intracellular signaling partners. Structural studies have revealed that GPCRs can exist in multiple conformational states, including inactive, active, and intermediate states. The inactive state represents the receptor in the absence of ligand binding, while the active state corresponds to the conformational changes induced by ligand binding which facilitates downstream signaling. Intermediate states capture the transitional phases between inactive and active conformations, providing a comprehensive understanding of the activation process as a whole.
GPCRs have an orthosteric binding site where native ligands bind in order to initiate the transition to an active state through rearrangement of the 7TM bundle. Native ligands and drug molecules which bind the orthosteric site can act as either agonists to directly activate the receptor, inverse agonists to suppress receptor activity below the basal level, or antagonists which bind to the orthosteric site to compete with other molecules but have no intrinsic effect[3]. Several allosteric binding sites have also been identified in GPCRs, presenting further targets for drug development beyond the orthosteric site. For instance, the opioid receptor family includes mu µ, delta δ, and kappa κ subtypes each have distinct ligand preferences and signaling profiles. Structural comparisons between these subtypes have unveiled the subtle variations in their binding pockets, explaining the subtype-specific effects of opioid ligands. Such insights are invaluable for designing selective drugs with reduced side effects.
Case Study: the Structures of GLP-1R
The Glucagon-like peptide-1 receptor (GLP-1R) is a GPCR that plays a critical role in glucose homeostasis. It has recently gained widespread attention as the target of a new class of drugs which improve type 2 diabetes treatment and promote weight loss to combat obesity (Ozempic®, Wegovy®, and Mounjaro®). As of this writing in October 2024, fifty structures of GLP-1R have been deposited in the Protein Data Bank, thirty-five of which were determined by cryo-EM and fifteen by x-ray crystallography. The majority of the cryo-EM structures are of full-length GLP-1R in complex with the trimeric g-proteins, and the x-ray crystallography structures are mostly of GLP-1R alone or a fragment of GLP-1R.
Most of the deposited structures are in complex with native ligands or potential drugs that bind to the orthosteric site as agonists to promote activation. A comparison between the ligand-bound structure and ligand-free GLP-1R reveals that ligands first bind to the ECD of GLP-1R to induce a subtle conformational change which makes the orthosteric pocket accessible[4]. The ligand can then bind at the orthosteric site to activate a signaling pathway which promotes insulin secretion and decreased appetite.
A few of the deposited structures have antibody fragments such as fabs or nanobodies bound as an alternative modality for modulating GLP-1R function. Most antibodies that target GLP-1R act as antagonists to prevent GLP-1 binding in individuals with hypoglycemia. These antibodies block the GLP-1 binding site of the ECD directly and thereby act as a competitive antagonist of native GLP-1[5].
In addition to elucidating the mechanism of activation or inhibition, structural studies of drug-bound GLP-1R have been critical for the development of therapeutics which are easily administered and have prolonged physiological effects. These insights into agonist and antagonist binding from experimentally determined structures deepen our understanding of drug interactions, paving the way for more effective and targeted GLP-1R therapeutics.
Contact us to see if your GPCR is suitable for our four week sample-to-structure workflow.
Citations & References
1. Yang, D., Zhou, Q., Labroska, V. et al. G protein-coupled receptors: structure- and function-based drug discovery. Sig Transduct Target Ther 6, 7 (2021). https://doi.org/10.1038/s41392-020-00435-w
2. Kee, T.R., Khan, S.A., Neidhart, M.B. et al. The multifaceted functions of β-arrestins and their therapeutic potential in neurodegenerative diseases. Exp Mol Med 56, 129–141 (2024). https://doi.org/10.1038/s12276-023-01144-4
3. Weis WI, Kobilka BK. The Molecular Basis of G Protein-Coupled Receptor Activation. Annu Rev Biochem. 2018 June 20;87:897-919. doi: 10.1146/annurev-biochem-060614-033910. PMID: 29925258; PMCID: PMC6535337.
4. Wu, F., Yang, L., Hang, K. et al. Full-length human GLP-1 receptor structure without orthosteric ligands. Nat Commun 11, 1272 (2020). https://doi.org/10.1038/s41467-020-14934-5
5. Hennen, S., Kodra, J., Soroka, V. et al. Structural insight into antibody-mediated antagonism of the Glucagon-like peptide-1 Receptor. Sci Rep6, 26236 (2016). https://doi.org/10.1038/srep26236
.webp)
Welcome to Finsweet's accessible modal component for Webflow Libraries. This modal uses Webflow Interactions to open and close. It is accessible through custom attributes and custom JavaScript added in the embed block of the component. If you're interested in how this is built, check out the Attributes documentation page for this modal component.
Infographic Available for Download
Explore GPCR structure, function, and their critical role in drug targeting, as well as how a structure based approach using cryo-EM can enhance GPCR targeting.
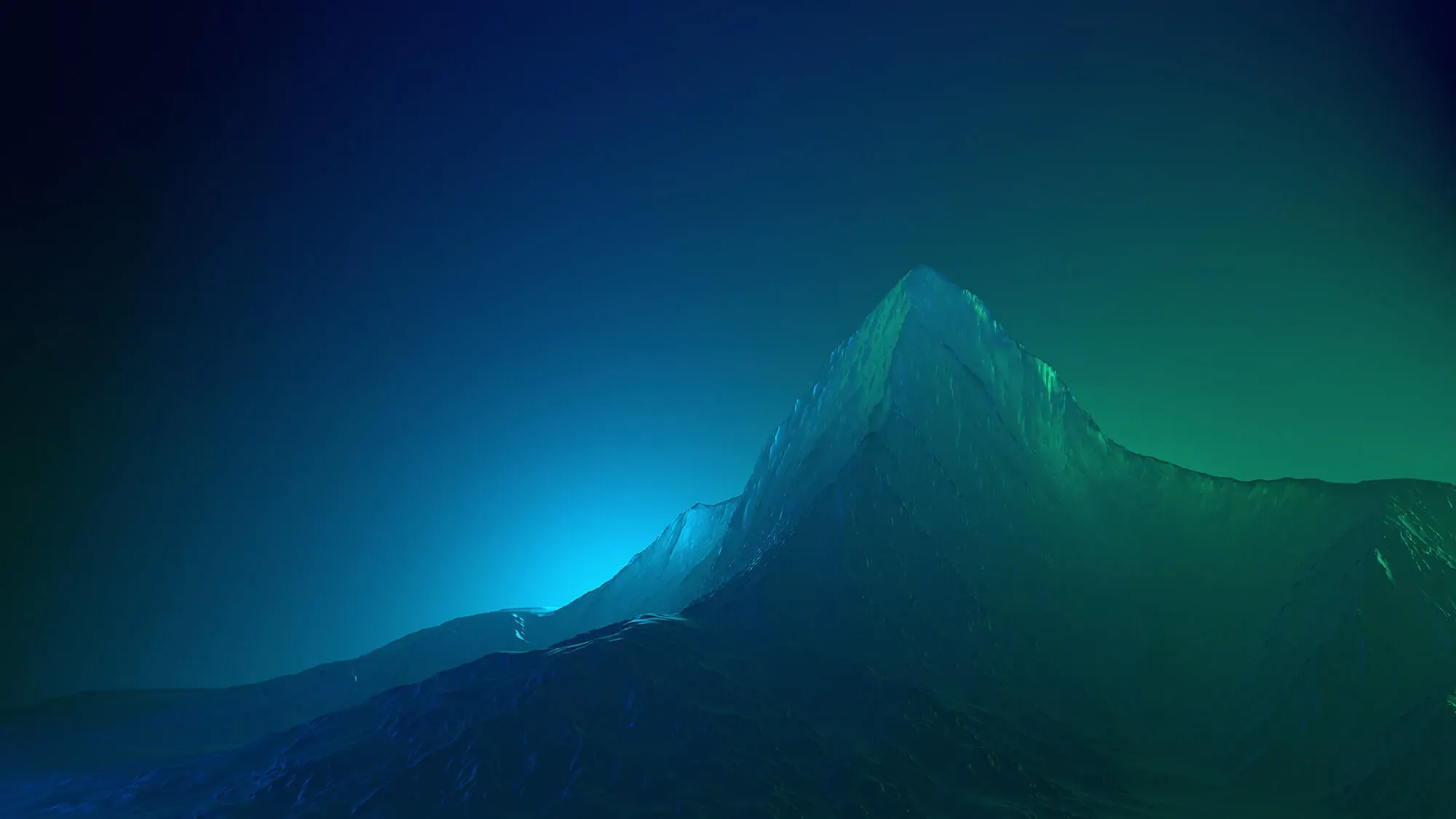